Abstract
Background:
Multiple sclerosis (MS) is an autoimmune disease in the central nervous system (CNS) that affects the development and physiological function of the brain and causes memory impairment.Objectives:
Due to the anti-inflammatory and antioxidant role of levothyroxine (L-T4) on myelin production and adult cerebral function, this study aimed to evaluate the effects of L-T4 on the improvement of cognitive deficits and cerebral inflammation.Methods:
Forty Wistar rats were randomly divided into five groups: (1) sham, (2) L-T4, (3) MS, (4) MS receiving L-T4, and (5) Betaferon. For MS induction, lysolecithin was injected into the CA1 of the hippocampus. Rats received L-T4 intraperitoneally at a dose of 100 μg/kg in the second and fourth groups. The shuttle box and Morris water maze tests were used to investigate passive avoidance and spatial memory, respectively. Also, the hippocampal concentrations of total antioxidant capacity (TAC), malondialdehyde (MDA), tumor necrosis factor-alpha (TNF-α), and c-reactive protein (CRP) were measured to investigate molecular changes.Results:
Path length (P < 0.001, P = 0.0015, and P = 0.002 on days 1 to 3, respectively) and latency time (P < 0.001 on the second and third days) increased, but the speed of movements (P < 0.001) and time spent in goal quarter decreased in MS-induced groups (P < 0.001). Treatment with L-T4 for 14 days significantly reversed path length and speed (P < 0.001 and P = 0.0315 on the second and third days), latency time (P < 0.01), speed (P < 0.001 and P = 0.0038 on the second and third days), and time spent in goal quarter (P = 0.1203) in the MS group. The hippocampal concentrations of MDA (P = 0.0010), TNF-α (P = 0.0251), and CRP (P = 0.0065) were significantly lower in the MS group treated with L-T4 than in the MS group. Also, the hippocampal concentration of TAC was significantly increased (P = 0.0375) in the MS group receiving L-T4.Conclusions:
It seems that treatment with L-T4 can prevent cognitive impairment caused by MS induction. Ameliorative effects of L-T4 may be due to the reduction of inflammation and oxidative stress. Therefore, L-T4 can be used as an effective agent in the treatment of MS.Keywords
Multiple Sclerosis Levothyroxine Cognition Oxidative Stress Inflammation
1. Background
Multiple sclerosis (MS) is a chronic autoimmune inflammatory disease of the central nervous system (CNS). It affects the myelin sheet of the nerve fibers and destroys them with the T and B cell function (1). Around 2.5 million people are affected by MS across the world (2). In MS, inflammatory cytokine cascades are activated, and neural fibers myelin sheets are degenerated (3). Recovery of myelin by oligodendrocyte precursor cells is the only efficient treatment for MS (4). Even though symptoms differ between MS patients, most patients experience at least one of the symptoms, including visual disturbances, tiredness, muscle cramps, depression, paralysis, vertigo, tremors, urinary incontinence, cognitive problems, and imbalance (5, 6).
The hippocampus is one of the main sites involved in learning and memory. This area is prone to neurological diseases due to its sensitivity to oxidative damage. On the other hand, cognitive impairment is seen in more than 65% of MS patients. Cognitive deficits have been recognized as one of the disabling clinical complications of MS. The most common cognitive disorder in these patients is a decrease in the speed of information processing and memory loss (7). Considering the high vulnerability of the hippocampus and its ability to regenerate, investigating the mechanisms involved in this process can provide a good study framework (3).
Studies have shown that tumor necrosis factor-alpha (TNF-α), which causes myelin damage (8), increases in patients with MS (9). Ozenci et al. showed that the number of TNF-α secreting cells is higher in patients with MS than in healthy individuals (10). Consequently, reducing this cytokine is invaluable in controlling MS (11, 12). Increased oxidative stress plays a significant role in the pathogenesis of neurodegenerative diseases (13). Malondialdehyde (MDA) as an index of lipid peroxidation and total antioxidant capacity (TAC) as an antioxidant index are considered indicators of oxidative stress (14). Based on the evidence, MDA and TAC concentrations vary greatly from their normal values in MS patients (15).
Also, MS patients suffer from autoimmune thyroid disease (1). Triiodothyronine (T3) and thyroxine (T4), as thyroid hormones (TH), are critical for the growth and functioning of the CNS. They are involved in the regular differentiation of neurons and the preservation of optimal cognitive ability in various conditions (16). The proliferation and development of oligodendrocytes (OLs) precursors into their final products, which produce myelin sheets, are affected by TH concentration (17). Besides, THs play a vital role in the development of the nervous system from prenatal to adulthood (18). Furthermore, THs play a vital role in the development and migration of OLs, which are responsible for myelination (11). Research has indicated a strong correlation between demyelinating diseases of the CNS and THs (13). It has been proposed that treatments involving THs could be beneficial for memory impairments in MS patients (15).
2. Objectives
This study examined the impact of L-T4 medication on cognitive deficits in an experimental model of MS.
3. Methods
3.1. Drugs: Lysolecithin, levothyroxine, and Betaferon
All reagents were of analytical purity. The assay kits for c-reactive protein (CRP), TAC, and MDA were purchased from Teb Pazhouhan Razi (TPR). Also, TNF-α kits were purchased from Diaclone (France).
3.2. Animals
Forty male Wistar rats (prepared by Animal Care and Breeding Center, Zahedan University of Medical Sciences, Zahedan, Iran) were used in this study. Rats (four per cage) were maintained in the laboratory at 23 ± 2°C with a relative humidity of 25% in a 12 h light/dark cycle (light on at 7:00 a.m.) for a week before the experiment. Animals had free access to water and standard laboratory feed. All experimental protocols were performed according to the regulations of the Ethics Committee of the Zahedan University of Medical Sciences, Zahedan, Iran (IR.ZAUMS.REC.1396.163).
3.3. Experimental Design
Animals were randomly divided into five groups: (1) sham group, for which stereotaxic surgery was performed, and a Hamilton needle was inserted into the skull, without any other treatment; (2) levothyroxine (L-T4) group, for which L-T4 at a dose of 100 μg/kg dissolved in 0.2 mL of normal saline was injected once a day for ten consecutive days; (3) MS group, for which the animals underwent stereotactic surgery and insertion of a guide cannula in the CA1 region of the hippocampus, followed by injecting 2 μL of lysolecithin (LPC) dissolved in 0.9% saline into the guide cannula for induction of demyelination; (4) MS + L-T4 group, for which rats received 2 μL of LPC, followed by L-T4 treatment; and (5) positive control (MS + Beta) group, for which rats received 2 μL of LPC, followed by Betaferon treatment. The vial of Betaferon was dissolved in 2 mL of distilled water, and subcutaneous doses were injected at 0.25 μg/kg for 10 days. All procedures were performed between 8:00 and 12:00 a.m. The experimental design is shown in Figure 1.
Parameters and time intervals in the experimental design
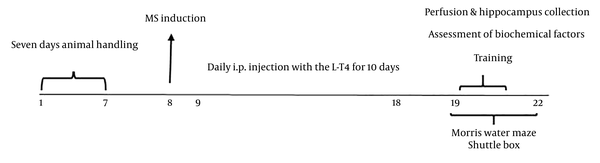
3.4. Passive Avoidance Test
A shuttle box containing two sections, a light chamber (20 × 20 × 30 cm) and a dark chamber (20 × 20 × 30 cm) was provided. An opening guillotine door separated the two compartments (6 × 6 cm). The description of the experiment is found elsewhere (19, 20). The initial latency (IL) on the third day and the step through latency (STL) on the fourth day were recorded as indices of the passive avoidance memory test (19).
3.5. Morris Water Maze
Spatial memory was evaluated by the Morris water maze (MWM) apparatus. The description of the experiment is presented elsewhere (19, 21). Briefly, a black circular pool (140 cm in diameter and 70 cm in height) was filled with tap water (24 ± 1°C) to a depth of 60 cm and was positioned in a room comprising extra maze visual cues. A hidden circular platform (12 cm in diameter) was submerged 1.5 cm beneath the surface of the water and located in the center of the southeast quadrant. Several parameters, for example, latency to find the hidden platform, path length, swimming speed, and time spent in the target quadrant, were recorded (14).
3.6. Measurement of CRP and TNF-α Concentrations
At the end of the behavioral tests, all rats were euthanized with a lethal dose of ketamine (100 mg/kg) and decapitated. Cerebral tissue was completely removed from the skull and quickly placed on ice. The hippocampus of rats was carefully separated from the rest of the brain under a stereoscope. After washing with saline solution and tris buffer, it was homogenized with a homogenizer at 5,000 rpm for 5 minutes (22). The homogenized solution was centrifuged, and phenyl methyl sulfonyl fluoride solution was used as a protease inhibitor. After centrifugation, the supernatant was separated with a sampler. Then, the tissue concentrations of CRP and TNF-α concentrations in the hippocampus were measured using ELISA kits (ZellBio Biotechnology Company, Lonsee, Germany), according to the manufacturer's instructions (23).
3.7. Measurement of Malondialdehyde and Total Antioxidant Capacity Concentrations
Malondialdehyde and TAC concentrations were measured by MDA and TAC ELISA kits according to the manufacturer's instructions (Teb Pazhouhan Razi) (21).
3.8. Statistical Analysis
Statistical analysis was done using the Graph Pad Prism 7.0 software. Data on MDA, TAC, CRP, and TNF-α concentration were analyzed by one-way ANOVA. However, shuttle box and MWM data were analyzed by one-way repeated-measures ANOVA, followed by Tukey's post hoc test. The results were presented as the mean ± SEM and regarded as significant at P values less than 0.05.
4. Results
4.1. Comparison of Initial Latency and Step-Through Latency in Passive Avoidance Test
Figure 2 shows IL and STL measurements in the passive avoidance test in all groups. The IL time to move from the light to the dark compartment of the shuttle box was similar in all groups before exposure to electrical stimulation. This variable showed slightly higher in the MS group, but it was not significant. Also, STL after 24 h was significantly lower in the MS group than in the sham group (P < 0.001). A 100 μg/kg of L-T4 (P < 0.001) and 0.25 μg/kg of Betaferon (P < 0.001) could increase STL significantly compared with the MS group.
Comparison of initial latency and step-trough latency in the passive avoidance test in the studied groups (n = 8). One-way repeated-measures ANOVA, followed by Tukey's post hoc test. *** P < 0.001 multiple sclerosis (MS) vs. sham, ### P < 0.001 MS + Levo and MS + Beta vs. MS.
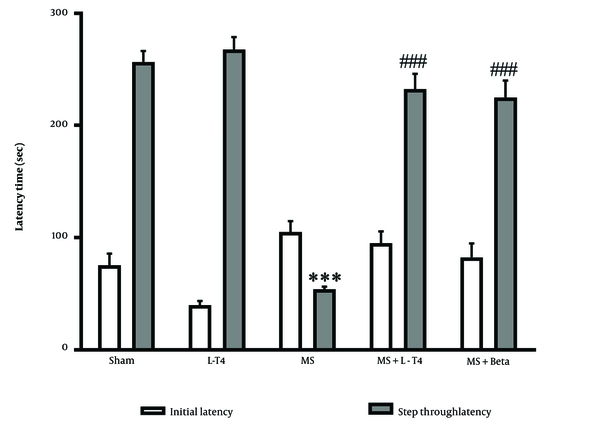
4.2. Comparison of Mean Latency Time to Find a Hidden Platform in the MWM Test
The average latency time to find the hidden platform was significantly higher in the MS group than in the sham group (*** P < 0.001). This parameter was significantly lower in the MS group treated with L-T4 (## P = 0.0071 and ## P = 0.0086 on the second and third days, respectively) compared to the MS group (Figure 3).
Comparison of mean swimming latency time to find a hidden platform in the Morris water maze, mean ± SEM (n = 8). One-way repeated-measures ANOVA, followed by Tukey's post hoc test). *** P < 0.001 multiple sclerosis (MS) vs. sham, # P < 0.05, ## P < 0.001 MS + Levo vs. MS.
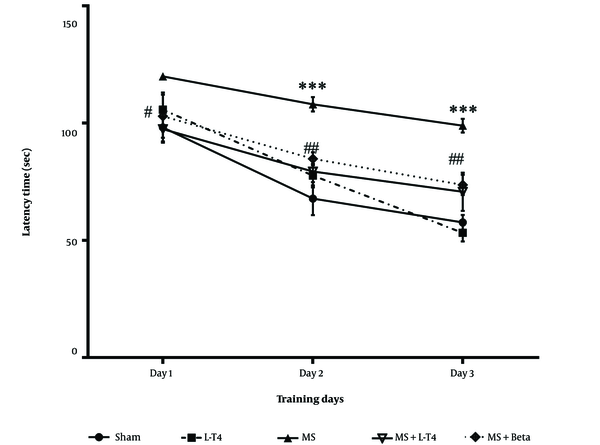
4.3. Comparison of the Mean Distance Traveled to Find the Hidden Platform in the MWM Test
The effect of LPC on traveled distance during acquisition trials is shown in Figure 4. Data analysis using one-way repeated-measures ANOVA showed that the distance traveled was significantly higher in the group treated with LPC on the first, second, and third days of training than in the sham group (P < 0.001, P = 0.0013, and P = 0.0002, respectively). The average distance traveled was significantly lower in the MS + Levo group than in the MS group on the second and third days (P = 0.0188 and P = 0.0004, respectively) during the three days of the acquisition trial. The distance traveled was not significantly different between the MS + Levo group and the MS+ Beta group.
Comparison of mean distance traveled to find the hidden platform in the Morris water maze (MWM) test during training days in the studied groups, mean ± SEM (n = 8). One-way repeated-measures ANOVA, followed by Tukey's post hoc test. ** P < 0.01, *** P < 0.001 multiple sclerosis (MS) vs. sham, # P < 0.05, ## P < 0.01, ### P < 0.001 MS + Levo and MS + Beta vs. MS.
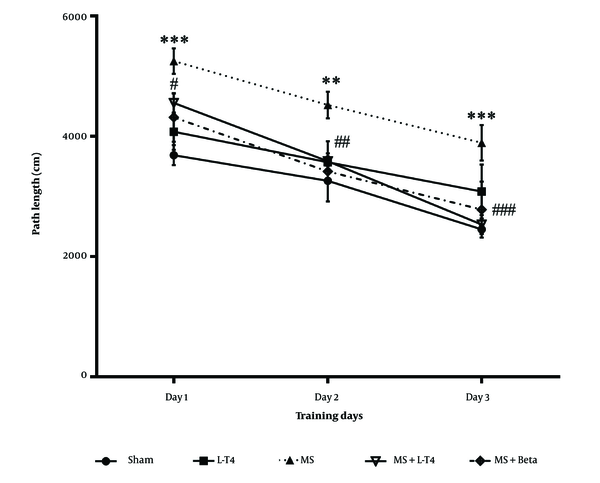
4.4. Comparison of the Mean Speed of Movement to Find the Hidden Platform on Training Days
The effect of LPC on speed during acquisition trials is shown in Figure 5. A one-way repeated-measures ANOVA and Tukey post-hoc tests showed that the speed of movement to find the hidden platform was significantly lower in the MS group on the first, second, and third days of training than in the sham group (P < 0.001). The mean speed was significantly higher in the MS + Levo group than in the MS group on the second and third days during the three days of acquisition trials (P = 0.0038, P < 0.001).
Comparison of average speed of movement to find the hidden platform in Morris water maze (MWM) test, mean ± SEM (n = 8). One-way repeated-measures ANOVA, followed by Tukey's post hoc test. *** P < 0.001 multiple sclerosis (MS) vs. sham, ## P < 0.01, ### P < 0.001 MS + Levo and MS + Beta vs. MS.
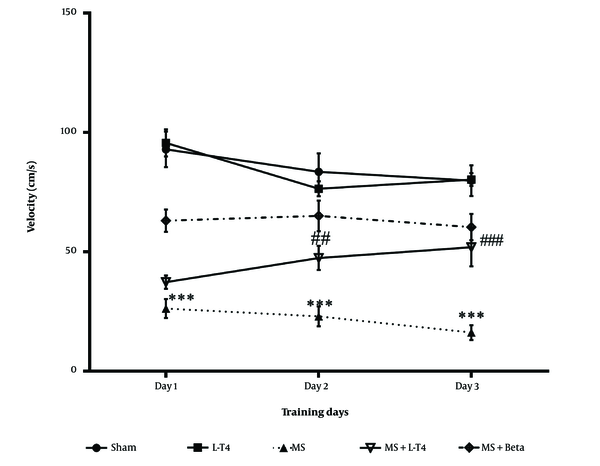
4.5. Comparison of the Time Spent in the Target Quadrant on the Test Day in the MWM Test
The probe trial was conducted 24 hours after the last acquisition day. One-way ANOVA demonstrated significant differences among groups. Tukey's post hoc test revealed that the MS group spent significantly less time than the sham group in the target quadrant (P < 0.001) (Figure 6). The mean time spent in the target quadrant was significantly higher in the MS + Levo (P = 0.0399) and MS + Betaferon groups (P = 0.0048) than in the MS group in the probe trial.
Comparison of the time spent in the target quadrant on the test day in the Morris water maze (MWM) test. *** P < 0.01 multiple sclerosis (MS) vs. sham, # P < 0.05, ## P < 0.01 MS + Levo and MS + Beta vs. MS.
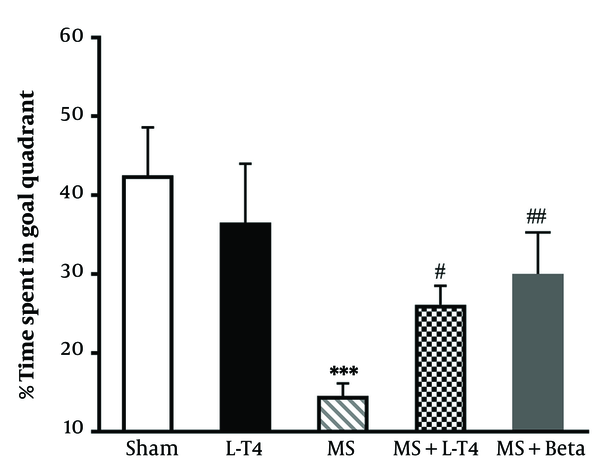
4.6. Comparison of CRP Concentrations in Hippocampus Tissue
The concentrations of CRP were significantly higher (P < 0.001) in the MS group than in the sham group. Also, this parameter was significantly lower in the MS + Levo group than in the MS group (P = 0.0065; Figure 7).
Comparison of the c-reactive protein (CRP) concentrations in homogenous hippocampus fluid in the studied groups (n = 8). *** P < 0.001 multiple sclerosis (MS) vs. sham, ## P < 0.01 MS + Levo and MS+ Beta vs. MS. Comparisons were made with a one-way ANOVA, followed by a Tukey post hoc test.
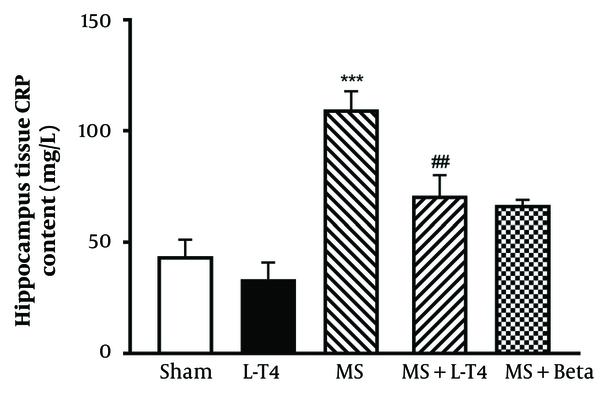
4.7. Comparison of TNF-α Concentrations in Hippocampus Tissue
The mean hippocampal TNF-α concentration was significantly higher in the MS group than in the sham group (P = 0.0005). The values were significantly lower in the groups treated with L-T4 (P = 0.0251) and Betaferon (P = 0.0003) than in the MS group (Figure 8).
Comparison of tumor necrosis factor-alpha (TNF-α) concentrations in homogeneous hippocampus fluid in the studied groups (n = 8). *** P < 0.001 multiple sclerosis (MS) vs. sham, # P < 0.05; ### P < 0.001 MS + Levo vs. MS. Comparisons were made with a one-way ANOVA, followed by a Tukey post hoc test.
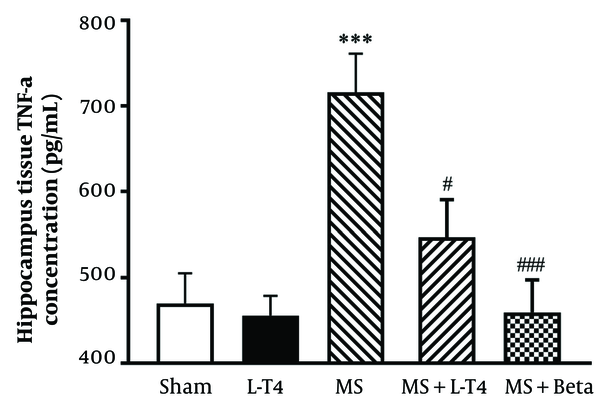
4.8. Comparison of TAC Concentrations in Hippocampus Tissue
The average concentration of TAC in the hippocampus is shown in Figure 9. Hippocampal TAC concentration was significantly (*** P = 0.0008) lower in the MS group than in the sham group. Also, TAC was significantly higher in MS groups treated with L-T4 (# P = 0.0375) and Betaferon (### P = 0.0008) than in the MS group.
Comparison of total antioxidant capacity (TAC) concentrations in homogeneous hippocampus fluid in the studied groups (n = 8). *** P < 0.001 multiple sclerosis (MS) vs. sham, # P < 0.05; ### P < 0.001 vs. MS, comparisons were made with a one-way ANOVA, followed by a Tukey post hoc test.
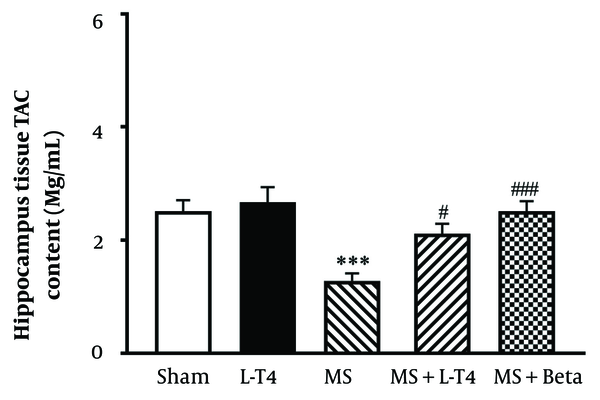
4.9. Comparison of MDA Concentrations in Hippocampus Tissue
The average concentration of MDA in the hippocampus is shown in Figure 10. The hippocampal MDA concentration was significantly higher in the MS group than in the sham group (*** P < 0.001). Also, the MDA concentrations were significantly lower in the MS groups treated with L-T4 (### P = 0.0010) and Betaferon (### P < 0.001) than in the MS group.
Comparison of malondialdehyde (MDA) concentrations in homogeneous hippocampus fluid in the studied groups (n = 8). *** P < 0.001 multiple sclerosis (MS) vs. sham, ### P < 0.001 vs. MS. Comparisons were made with a one-way ANOVA, followed by a Tukey post hoc test.
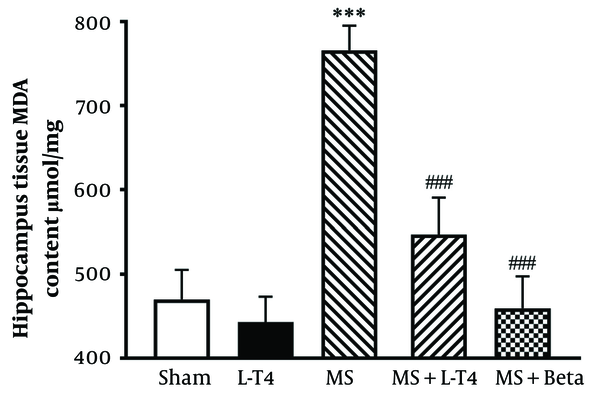
5. Discussion
The current study confirmed that the administration of LPC has a negative impact on the learning and memory of rats, as demonstrated by the results of the MWM and PAT tests. Demyelination was induced in this study by injecting LPC into the CA1 region of the hippocampus. The study revealed that injecting 2 µL of LPC directly into the CA1 region of the hippocampus impairs the learning and spatial memory performance of the hippocampus. The PAT test, conducted in a shuttle box, did not show any significant difference between the groups in terms of IL, which indicates the animals' ability to learn behavior. However, STL, which represents the animals' ability to consolidate memory information and recall it, was significantly improved in the MS group treated with L-T4 and interferon β (positive control) compared to the MS group. The MWM test is used to measure spatial memory. The distance and time taken to find the hidden platform were significantly increased, and the speed of movement to find the hidden platform was decreased in the MS group. The results indicated that injecting L-T4 into the hippocampus improved learning and memory impairment after LPC injection in the CA1 region of the hippocampus. In the MWM test, administering L-T4 reduced the time spent to find the hidden platform and increased the time spent in the target quadrant on the probe day. In contrast, injecting L-T4 at 100 µg/kg and Betaferon at 0.25 µg/kg reduced the concentration of MDA and increased TAC in the MS groups treated with L-T4 and Betaferon. It was also observed that TNFα and CRP levels increased in the MS group. However, in the MS group treated with L-T4 and Betaferon, TNF-α concentrations decreased.
The structural organization of the hippocampus is one of the most important gray matter regions of the CNS in MS (24). Cognitive impairment is a significant factor affecting the quality of life in individuals with MS. There is a consensus that the health of the hippocampus plays a crucial role in spatial memory (25). In contrast, damage to the hippocampus can result in spatial memory disorders. Dysfunctions in neurotransmitters and disturbances in GABA and adenosine concentrations, as well as redox systems in the CNS caused by THs dysfunction, can hinder neurogenesis and CNS growth (26). Therefore, maintaining stable concentrations of THs can help prevent the development of neurodegenerative diseases. Pharmacokinetic analyses have shown that L-T4 can cross the blood-brain barrier (27), which is usually impermeable to triiodothyronine (T3). Mostly, T3 in cerebral tissue is locally produced through the conversion from T4. Besides, L-T4 administration raises both serum and cerebral concentrations of free T4 and T3 (26).
Previous studies have demonstrated that injecting LPC into the CA1 region of the hippocampus leads to impairment in learning and memory (28). This study also observed learning and memory impairment in rats after LPC injection, consistent with previous findings. Administration of LPC causes neuronal loss in various parts of the hippocampus and results in cognitive impairment (29). Injecting LPC into the CA1 region of the hippocampus triggers microglia to produce pro-inflammatory cytokines (30). Besides, L-T4 can inhibit neuro-inflammation by suppressing the production of TNF-α and CRP. Also, L-T4 has neuroprotective effects and may have potential benefits for treating neuro-inflammation induced by MS. In addition, THs play a significant role in the organization and function of the brain throughout life (31). Although their effects during embryonic development are well-documented (32), their impacts on cerebral function in adults, particularly during puberty, are less understood (31).
Other studies showed that L-T4 plays an important role in the development of remyelination after injuries. The absence of these hormones causes a decrease in myelin production (33). Also, THs can enter brain tissues through the choroid carriers and affect all types of cells in this area (34). Myelination is a TH-dependent process and plays an important role in the production and development of OLs (35). Since remyelination needs initialization of the initial stages of myelination (36), remyelination in the MS may also require THs (37). In particular, L-T4 could prevent the damage caused by LPC injection in optic chiasma in the demyelination phase, indicating the role of THs in the destruction of inflammatory-induced myelin. THs are likely to affect myelin health by acting on immune cells (38). High concentrations of T4 suppress T cell transcription and greatly reduce the production of pro-inflammatory cytokines (39). Thus, THs retain the proliferation and differentiation of OLs progenitor cells into OPC by reducing pro-inflammatory cytokines (40).
The structure and organization of cellular skeletal proteins, as well as the extracellular matrix (41), affect the process of demyelination and remyelination. Several lines of evidence suggest that memory repair in rats treated with L-T4 suppresses gene expression of the beta-amyloid precursor protein. Also, THs stimulate neuronal proliferation and survival and increase the activation of the phosphoinositide-3 kinase/protein kinase-B (PI3K/Akt) pathway. Subsequently, these functions of L-T4 could be associated with neuroprotection (42).
Furthermore, L-T4 has been shown to increase brain-derived neurotrophic factor (43). As a possible action mechanism, L-T4 improves memory by regulating cholinergic function, protecting the brain against damaging effects of free radicals, and protecting hippocampal neurons from apoptosis (44). As known, THs participate in scavenging oxygen free radicals by increasing non-enzymatic antioxidant molecules (45). This group of antioxidants increases the level of antioxidant enzymes such as superoxide dismutase (SOD), catalase, and glutathione peroxidase (46). They stimulate the unpaired mitochondrial protein, which is a non-enzymatic antioxidant molecule (47).
A significant increase in MDA concentrations in the MS group compared to the sham group showed that free radicals were produced during the disease period, and the incidence of lipid peroxidation was due to oxidative stress. In the MS group treated with L-T4, MDA concentrations showed a significant decrease compared to the MS group. This finding suggests that L-T4 may affect oxidative stress. The antioxidant properties of L-T4 reduce oxidative damage and lipid peroxidation, thereby reducing the MDA index. A decrease in MDA and an increase in SOD levels after 6 months of treatment with L-T4 have been previously shown (48). This study showed that TNF-α and CRP concentrations increased in the MS group. Tumor necrosis factor-alpha, as a strong stimulant, reduces interleukin-6 production, which is a powerful stimulant for the production of CRP (49).
On the other hand, the concentration of CRP in the MS group receiving L-T4 decreased significantly. Tumor necrosis factor-alpha plays a dual role in patients with MS; it increases with the degradation of myelin; on the other hand, this agent plays a protective role in the nerves by increasing the proliferation of oligodendrocytes and stimulating the reuptake of myelin. One possible explanation may be the existence of two TNF-α receptors for different signaling paths (P55, P57) (50). The neuronal protective function of the TNF-α P57 receptor by induction of SOD protects neurons from reactive oxygen species (ROS) (51).
Fu et al. declared that L-T4 is effective in reversing learning and memory deficits in a mouse model of beta-amyloid-induced Alzheimer's disease (44). In examining the level of cholinesterase, it has been shown that the injection of L-T4 into rats leads to an increase in the cholinergic function of the hippocampus (27). These results suggest a direct connection between two signaling systems. The cholinergic signal in the CNS was very vital for cognitive processes (52). Thyroid hormones control the transcription of the genes and the expression of signaling secondary proteins (53). These are important for many metabolic processes, including vital signaling systems for learning, memory, and synapse molding, including reelin (43), choline acetyltransferase receptors, and NMDA receptors (53). Thus, the ability of THs to control gene expression can help cell signaling cascades for learning and memory. More studies are needed to find other pathways explaining the effects of L-T4 on cognitive deficits caused by MS.
5.1. Conclusions
The present study showed that the injection of LPC in the CA1 region of the hippocampus causes cognition decline and neuronal damage, followed by increased inflammation and free radicals. Treatment with L-T4 improved passive avoidance memory through its antioxidant activity. Also, L-T4 ameliorated spatial memory by decreasing the concentration of hippocampal inflammatory cytokines such as TNF-α and CRP. Treatment with L-T4 improved passive avoidance and spatial memories because of its anti-inflammatory and antioxidant activity, increased the level of TAC of the hippocampus, and reduced MDA, TNFα, and CRP concentrations.
References
-
1.
Jalkanen A, Saraste M, Gfeller A, Surcel HM, Airas L. Increased thyroid autoimmunity among women with multiple sclerosis in the postpartum setting. Mult Scler. 2013;19(13):1734-42. [PubMed ID: 23629943]. https://doi.org/10.1177/1352458513485148.
-
2.
Paintlia AS, Mohan S, Singh I. Combinatorial Effect of Metformin and Lovastatin Impedes T-cell Autoimmunity and Neurodegeneration in Experimental Autoimmune Encephalomyelitis. J Clin Cell Immunol. 2013;4(3):1000149. [PubMed ID: 24324917]. [PubMed Central ID: PMC3855339]. https://doi.org/10.4172/2155-9899.1000149.
-
3.
Babri S, Mehrvash F, Mohaddes G, Hatami H, Mirzaie F. Effect of intrahippocampal administration of vitamin C and progesterone on learning in a model of multiple sclerosis in rats. Adv Pharm Bull. 2015;5(1):83-7. [PubMed ID: 25789223]. [PubMed Central ID: PMC4352227]. https://doi.org/10.5681/apb.2015.011.
-
4.
Bribian A, Medina-Rodriguez EM, Josa-Prado F, Garcia-Alvarez I, Machin-Diaz I, Esteban PF, et al. Functional Heterogeneity of Mouse and Human Brain OPCs: Relevance for Preclinical Studies in Multiple Sclerosis. J Clin Med. 2020;9(6):1681. [PubMed ID: 32498223]. [PubMed Central ID: PMC7355819]. https://doi.org/10.3390/jcm9061681.
-
5.
Jahani GH, Firoozrai M, Matin Homaee H, Tarverdizadeh B, Azarbayjani MA, Movaseghi GR, et al. [The Effect of Continuous and Regular Exercise on Erytrocyte Antioxidative Enzymes Activity and Stress Oxidative in Young Soccer Players]. Razi J Med Sci. 2010;17(74):22-32. Persian.
-
6.
Omotoso GO, Gbadamosi IT, Afolabi TT, Abdulwahab AB, Akinlolu AA. Ameliorative effects of Moringa on cuprizone-induced memory decline in rat model of multiple sclerosis. Anat Cell Biol. 2018;51(2):119-27. [PubMed ID: 29984057]. [PubMed Central ID: PMC6026826]. https://doi.org/10.5115/acb.2018.51.2.119.
-
7.
Vilou I, Varka A, Parisis D, Afrantou T, Ioannidis P. EEG-Neurofeedback as a Potential Therapeutic Approach for Cognitive Deficits in Patients with Dementia, Multiple Sclerosis, Stroke and Traumatic Brain Injury. Life (Basel). 2023;13(2):365. [PubMed ID: 36836721]. [PubMed Central ID: PMC9966294]. https://doi.org/10.3390/life13020365.
-
8.
Fresegna D, Bullitta S, Musella A, Rizzo FR, De Vito F, Guadalupi L, et al. Re-Examining the Role of TNF in MS Pathogenesis and Therapy. Cells. 2020;9(10):2290. [PubMed ID: 33066433]. [PubMed Central ID: PMC7602209]. https://doi.org/10.3390/cells9102290.
-
9.
Mosayebi G, Ghazavi A, Aghili B, Mirshafiei A. [Immunomodulating activity of Aloe Vera in animal model of multiple sclerosis]. J Arak Uni Med Sci. 2009;12(3):109-15. Persian.
-
10.
Ozenci V, Kouwenhoven M, Huang YM, Kivisakk P, Link H. Multiple sclerosis is associated with an imbalance between tumour necrosis factor-alpha (TNF-alpha)- and IL-10-secreting blood cells that is corrected by interferon-beta (IFN-beta) treatment. Clin Exp Immunol. 2000;120(1):147-53. [PubMed ID: 10759776]. [PubMed Central ID: PMC1905623]. https://doi.org/10.1046/j.1365-2249.2000.01175.x.
-
11.
Robinson WH, Genovese MC, Moreland LW. Demyelinating and neurologic events reported in association with tumor necrosis factor alpha antagonism: by what mechanisms could tumor necrosis factor alpha antagonists improve rheumatoid arthritis but exacerbate multiple sclerosis? Arthritis Rheum. 2001;44(9):1977-83. [PubMed ID: 11592357]. https://doi.org/10.1002/1529-0131(200109)44:9<1977::AID-ART345>3.0.CO;2-6.
-
12.
Ribeiro CM, Oliveira SR, Alfieri DF, Flauzino T, Kaimen-Maciel DR, Simao ANC, et al. Tumor necrosis factor alpha (TNF-alpha) and its soluble receptors are associated with disability, disability progression and clinical forms of multiple sclerosis. Inflamm Res. 2019;68(12):1049-59. [PubMed ID: 31559449]. https://doi.org/10.1007/s00011-019-01286-0.
-
13.
Tarbali S, Khezri S, Heidari R. [Effect of Vitamin D3 on Improvement of Learning and Spatial Memory Following Demyelination induction in hippocampal CA1 Area of rat]. Physiol Pharmacol. 2014;17(4):449-60. Persian.
-
14.
Sedaghat G, Mirshekar MA, Amirpour M, Montazerifar F, Miri S, Shourestani S. Sub-chronic administration of brewed coffee on rat behavior and cognition and oxidative stress Alzheimer's disease model. Clin Nutr Exp. 2019;28:62-73. https://doi.org/10.1016/j.yclnex.2019.10.005.
-
15.
Saghebjoo M, Dehghani Firouzabadi M, Etesami M, Mahmudzadeh T. [Effect of Pilates Training on Serum Levels of Brain-Derived Neurotrophic Factor, Malondialdehyde and Total Antioxidant Capacity in Women with Multiple Sclerosis]. Sport Physiol. 2016;8(30):143-58. Persian. https://doi.org/10.22089/spj.2016.775.
-
16.
van Vliet NA, van Heemst D, Almeida OP, Asvold BO, Aubert CE, Bae JB, et al. Association of Thyroid Dysfunction With Cognitive Function: An Individual Participant Data Analysis. JAMA Intern Med. 2021;181(11):1440-50. [PubMed ID: 34491268]. [PubMed Central ID: PMC8424529]. https://doi.org/10.1001/jamainternmed.2021.5078.
-
17.
Kuhn S, Gritti L, Crooks D, Dombrowski Y. Oligodendrocytes in Development, Myelin Generation and Beyond. Cells. 2019;8(11):1424. [PubMed ID: 31726662]. [PubMed Central ID: PMC6912544]. https://doi.org/10.3390/cells8111424.
-
18.
Bye CM, Hong NS, Moore K, Deibel SH, McDonald RJ. The effects of pool shape manipulations on rat spatial memory acquired in the Morris water maze. Learn Behav. 2019;47(1):29-37. [PubMed ID: 29520732]. https://doi.org/10.3758/s13420-018-0319-0.
-
19.
Mirshekar MA, Miri S, Shahraki A. A Survey of the Effects of Diosmin on Learning and Memory Following the Use of Paraquat Herbicide Poisoning in a Model of Rats. Shiraz E-Med J. 2020;21(5):e94143. https://doi.org/10.5812/semj.94143.
-
20.
Keikhaei F, Mirshekar MA, Shahraki MR, Dashipour A. Antiepileptogenic effect of myricitrin on spatial memory and learning in a kainate-induced model of temporal lobe epilepsy. Learn Motiv. 2020;69:101610. https://doi.org/10.1016/j.lmot.2019.101610.
-
21.
Mirshekar MA, Shahraki M, Najafi R, Shabani S. The ameliorative effects of myricetin on neurobehavioral activity, electrophysiology, and biochemical changes in an animal model of traumatic brain injury. Learn Motiv. 2019;68:101597. https://doi.org/10.1016/j.lmot.2019.101597.
-
22.
Amirpour M, Mirshekar MA, Sedaghat G, Montazerifar F, Shourestani S, Arabmoazzen S, et al. The effects of green tea on cognitive impairments in the rat model of Alzheimer's disease: protection against inflammatory and oxidative damage. Nutr Neurosci. 2022;25(12):2659-67. [PubMed ID: 34802394]. https://doi.org/10.1080/1028415X.2021.2003946.
-
23.
Naghizadeh M, Mirshekar MA, Montazerifar F, Saadat S, Shamsi Koushki A, Jafari Maskouni S, et al. Effects of quercetin on spatial memory, hippocampal antioxidant defense and BDNF concentration in a rat model of Parkinson's disease: An electrophysiological study. Avicenna J Phytomed. 2021;11(6):599-609. [PubMed ID: 34804897]. [PubMed Central ID: PMC8588960]. https://doi.org/10.22038/AJP.2021.18526.
-
24.
Goudarzvand M, Javan M, Mirnajafi-Zadeh J, Mozafari S, Tiraihi T. Vitamins E and D3 attenuate demyelination and potentiate remyelination processes of hippocampal formation of rats following local injection of ethidium bromide. Cell Mol Neurobiol. 2010;30(2):289-99. [PubMed ID: 19768531]. https://doi.org/10.1007/s10571-009-9451-x.
-
25.
Ziehn MO, Avedisian AA, Dervin SM, Umeda EA, O'Dell TJ, Voskuhl RR. Therapeutic testosterone administration preserves excitatory synaptic transmission in the hippocampus during autoimmune demyelinating disease. J Neurosci. 2012;32(36):12312-24. [PubMed ID: 22956822]. [PubMed Central ID: PMC3571760]. https://doi.org/10.1523/JNEUROSCI.2796-12.2012.
-
26.
Kaptan Z, Üzüm G. The Role of Adult Hippocampal Neurogenesis in Learning and Memory Function. Turk J Neurol. 2016;22(4):149-55. https://doi.org/10.4274/tnd.68889.
-
27.
Fu A, Zhou R, Xu X. The synthetic thyroid hormone, levothyroxine, protects cholinergic neurons in the hippocampus of naturally aged mice. Neural Regen Res. 2014;9(8):864-71. [PubMed ID: 25206902]. [PubMed Central ID: PMC4146262]. https://doi.org/10.4103/1673-5374.131602.
-
28.
Tarbali S, Khezri S. Vitamin D3 attenuates oxidative stress and cognitive deficits in a model of toxic demyelination. Iran J Basic Med Sci. 2016;19(1):80-8. [PubMed ID: 27096068]. [PubMed Central ID: PMC4823620].
-
29.
Roux CM, Leger M, Freret T. Memory Disorders Related to Hippocampal Function: The Interest of 5-HT(4)Rs Targeting. Int J Mol Sci. 2021;22(21):12082. [PubMed ID: 34769511]. [PubMed Central ID: PMC8584667]. https://doi.org/10.3390/ijms222112082.
-
30.
Kovacsova M, Barta A, Parohova J, Vrankova S, Pechanova O. Neuroprotective mechanisms of natural polyphenolic. Activitas Nervosa Superior Rediviva. 2010;52(3):181-6.
-
31.
Remaud S, Gothie JD, Morvan-Dubois G, Demeneix BA. Thyroid hormone signaling and adult neurogenesis in mammals. Front Endocrinol (Lausanne). 2014;5:62. [PubMed ID: 24808891]. [PubMed Central ID: PMC4009442]. https://doi.org/10.3389/fendo.2014.00062.
-
32.
Bernal J. Thyroid hormone receptors in brain development and function. Nat Clin Pract Endocrinol Metab. 2007;3(3):249-59. [PubMed ID: 17315033]. https://doi.org/10.1038/ncpendmet0424.
-
33.
Dugas JC, Ibrahim A, Barres BA. The T3-induced gene KLF9 regulates oligodendrocyte differentiation and myelin regeneration. Mol Cell Neurosci. 2012;50(1):45-57. [PubMed ID: 22472204]. [PubMed Central ID: PMC4441621]. https://doi.org/10.1016/j.mcn.2012.03.007.
-
34.
Miller E, Mrowicka M, Malinowska K, Mrowicki J, Saluk-Juszczak J, Kędziora J. The effects of whole-body cryotherapy on oxidative stress in multiple sclerosis patients. J Therm Biol. 2010;35(8):406-10. https://doi.org/10.1016/j.jtherbio.2010.08.006.
-
35.
Calza L, Fernandez M, Giardino L. Cellular approaches to central nervous system remyelination stimulation: thyroid hormone to promote myelin repair via endogenous stem and precursor cells. J Mol Endocrinol. 2010;44(1):13-23. [PubMed ID: 19578096]. https://doi.org/10.1677/JME-09-0067.
-
36.
Miller RH, Mi S. Dissecting demyelination. Nat Neurosci. 2007;10(11):1351-4. [PubMed ID: 17965654]. https://doi.org/10.1038/nn1995.
-
37.
Tepavcevic V. Oligodendroglial Energy Metabolism and (re)Myelination. Life (Basel). 2021;11(3):238. [PubMed ID: 33805670]. [PubMed Central ID: PMC7998845]. https://doi.org/10.3390/life11030238.
-
38.
Rashidi B, Payghani C, Khani F, Rafieezadeh A, Alaei H, Reisi P. [The Effect of Levothyroxine on Lysolecithin-Induced Local Demyelination in Optic Chiasm of Male Rats]. J Isfahan Med Sch. 2017;35(437):789-95. Persian.
-
39.
Mancini A, Di Segni C, Raimondo S, Olivieri G, Silvestrini A, Meucci E, et al. Thyroid Hormones, Oxidative Stress, and Inflammation. Mediators Inflamm. 2016;2016:6757154. [PubMed ID: 27051079]. [PubMed Central ID: PMC4802023]. https://doi.org/10.1155/2016/6757154.
-
40.
Trentin AG. Thyroid hormone and astrocyte morphogenesis. J Endocrinol. 2006;189(2):189-97. [PubMed ID: 16648287]. https://doi.org/10.1677/joe.1.06680.
-
41.
Alizadeh A, Dyck SM, Karimi-Abdolrezaee S. Myelin damage and repair in pathologic CNS: challenges and prospects. Front Mol Neurosci. 2015;8:35. [PubMed ID: 26283909]. [PubMed Central ID: PMC4515562]. https://doi.org/10.3389/fnmol.2015.00035.
-
42.
Cao X, Kambe F, Yamauchi M, Seo H. Thyroid-hormone-dependent activation of the phosphoinositide 3-kinase/Akt cascade requires Src and enhances neuronal survival. Biochem J. 2009;424(2):201-9. [PubMed ID: 19747164]. https://doi.org/10.1042/BJ20090643.
-
43.
Sui L, Ren WW, Li BM. Administration of thyroid hormone increases reelin and brain-derived neurotrophic factor expression in rat hippocampus in vivo. Brain Res. 2010;1313:9-24. [PubMed ID: 20018181]. https://doi.org/10.1016/j.brainres.2009.12.010.
-
44.
Fu AL, Zhou CY, Chen X. Thyroid hormone prevents cognitive deficit in a mouse model of Alzheimer's disease. Neuropharmacology. 2010;58(4-5):722-9. [PubMed ID: 20045708]. https://doi.org/10.1016/j.neuropharm.2009.12.020.
-
45.
Villanueva I, Alva-Sanchez C, Pacheco-Rosado J. The role of thyroid hormones as inductors of oxidative stress and neurodegeneration. Oxid Med Cell Longev. 2013;2013:218145. [PubMed ID: 24386502]. [PubMed Central ID: PMC3872098]. https://doi.org/10.1155/2013/218145.
-
46.
Messarah M, Boulakoud MS, Boumendjel A, Abdennour C, El Feki A. The impact of thyroid activity variations on some oxidizing-stress parameters in rats. C R Biol. 2007;330(2):107-12. [PubMed ID: 17303537]. https://doi.org/10.1016/j.crvi.2006.11.004.
-
47.
Ighodaro OM, Akinloye OA. First line defence antioxidants-superoxide dismutase (SOD), catalase (CAT) and glutathione peroxidase (GPX): Their fundamental role in the entire antioxidant defence grid. Alexandria J Med. 2018;54(4):287-93. https://doi.org/10.1016/j.ajme.2017.09.001.
-
48.
Masullo LF, Magalhaes RA, Lemes RPG, de Almeida Filho TP, de Castro MF, Maia Filho PA, et al. Levothyroxine Replacement Improves Oxidative Status in Primary Hypothyroidism. Front Endocrinol (Lausanne). 2018;9:655. [PubMed ID: 30467493]. [PubMed Central ID: PMC6235903]. https://doi.org/10.3389/fendo.2018.00655.
-
49.
Sproston NR, Ashworth JJ. Role of C-Reactive Protein at Sites of Inflammation and Infection. Front Immunol. 2018;9:754. [PubMed ID: 29706967]. [PubMed Central ID: PMC5908901]. https://doi.org/10.3389/fimmu.2018.00754.
-
50.
Podbielska M, Banik NL, Kurowska E, Hogan EL. Myelin recovery in multiple sclerosis: the challenge of remyelination. Brain Sci. 2013;3(3):1282-324. [PubMed ID: 24961530]. [PubMed Central ID: PMC4061877]. https://doi.org/10.3390/brainsci3031282.
-
51.
Pozniak PD, White MK, Khalili K. TNF-alpha/NF-kappaB signaling in the CNS: possible connection to EPHB2. J Neuroimmune Pharmacol. 2014;9(2):133-41. [PubMed ID: 24277482]. [PubMed Central ID: PMC3959244]. https://doi.org/10.1007/s11481-013-9517-x.
-
52.
Hampel H, Mesulam MM, Cuello AC, Farlow MR, Giacobini E, Grossberg GT, et al. The cholinergic system in the pathophysiology and treatment of Alzheimer's disease. Brain. 2018;141(7):1917-33. [PubMed ID: 29850777]. [PubMed Central ID: PMC6022632]. https://doi.org/10.1093/brain/awy132.
-
53.
Sola E, Moyano P, Flores A, Garcia J, Garcia JM, Anadon MJ, et al. Cadmium-induced neurotoxic effects on rat basal forebrain cholinergic system through thyroid hormones disruption. Environ Toxicol Pharmacol. 2022;90:103791. [PubMed ID: 34968718]. https://doi.org/10.1016/j.etap.2021.103791.